- LEVITY
- Posts
- Antagonistic pleiotropy: Why aging is built into our genes
Antagonistic pleiotropy: Why aging is built into our genes
Some genes help us thrive early in life - then betray us later. Here's how evolution made that deal.

The Antagonistic pleiotropy hypothesis (in 2025)
The Antagonistic pleiotropy hypothesis is a key evolutionary hypothesis that partly explains why organisms age. It proposes that some genes offer benefits early in life - such as increased fertility or growth - but cause harm in later years, contributing to aging and disease. This trade-off helps explain why evolution preserves certain ”aging genes” despite their late-life costs.
💡 This article is part of LEVITY Knowledge, a series of explainers on longevity science and aging biology. LEVITY is a podcast and newsletter about unlimited lifespan and the future of medicine. Subscribe here for weekly insights on longevity biotech and the quest to solve aging.
Historical Context and Development
In 1951, British immunologist and Nobel laureate Peter Medawar proposed a theory that would reshape our understanding of aging: the accumulation of cell-level damage over time. The idea is surprisingly straightforward.
No matter the organism, a gene’s ”goal” is to propagate itself - and evolution, in a sense, is the process that enables it to do so. If a particular trait increases the odds of survival and reproduction, natural selection will tend to favor and preserve it. But once reproduction is complete, the gene has already succeeded in passing itself on. Evolution applies little pressure to eliminate harmful effects that only appear late in life - making aging and late-life disease its blind spot.
For most of evolutionary history, this wasn’t a major issue. We (and many other animals) often died young - victims of external threats - long before aging could take its toll. As Medawar put it, evolution exerts little pressure on mutations that only manifest after reproduction.

A somewhat faithful ChatGPT illustration of George C. Williams.
A few years later, another evolutionary biologist, George C. Williams, expanded on Medawar’s idea with a more elegant theory: antagonistic pleiotropy.
The roots of the antagonistic pleiotropy concept trace back, indirectly, to Gregor Mendel, the father of genetics. In his mid-1800s experiments with pea plants, Mendel noticed that certain traits appeared together - for example, white flowers were consistently associated with colorless seed coats and leaf buds, while purple flowers came with brown-gray seeds and reddish buds. While Mendel didn’t know it at the time, this hinted at what modern biology calls pleiotropy - the idea that a single gene can influence multiple traits.
Williams proposed that some genes may be beneficial early in life, helping us survive and reproduce, but harmful later, contributing to aging and disease. Evolution, he argued, is willing to make that trade-off.
This is the essence of the antagonistic pleiotropy hypothesis - a gene that’s helpful in youth but a liability in old age, preserved by evolution because of its early-life advantages.
How it Affect Humans and Animals
To be precise, J.B.S. Haldane, another British researcher, actually preceded both Medawar and Williams. As early as 1941, he speculated that late-onset genetic diseases like Huntington’s might persist in populations because their harmful effects appear after reproduction - offering an early glimpse of what would later be called the antagonistic pleiotropy hypothesis (though he didn’t use that term).
Huntington’s disease is an inherited neurological disorder that affects cognition, mood, and motor control. Symptoms typically don’t appear until between the ages of 30 and 50 - by which time many individuals have already had children. Some studies suggest that female carriers of the HTT mutation may have higher fertility than non-carriers, and there is also evidence that people with Huntington’s may have increased protection against certain cancers.
I have a personal connection to this topic.* I live with a hereditary blood condition called thalassemia, which is also sometimes cited as an example of antagonistic pleiotropy - though with one important difference. Like Huntington’s, thalassemia appears to offer both benefits and drawbacks, but the effect isn’t tied to age. Instead, it depends on which variant of the gene you inherit.
* Find out more about me and the origin story for this newsletter here.
In my case, I have beta-thalassemia minor, the heterozygous form of the disease. This means I carry two different versions of the gene, inherited from each parent. The more severe homozygous variant, beta-thalassemia major, arises when both copies of the gene carry the same mutation.
It’s no surprise I inherited the gene from my father - thalassemia is common in regions around the Mediterranean. In fact, my variant offers increased protection against malaria, which likely explains its evolutionary persistence.
That said, being born and raised in Sweden, my so-called mutant powers aren’t exactly superhero material. My version of the disease impairs my red blood cells’ ability to transport oxygen - something I wish I’d known as a teenager when my football coach kept criticizing my stamina. Then again, that argument falls a bit flat, since Zinedine Zidane also has thalassemia.
For those with the homozygous form, nature is far less kind. The malaria protection disappears, and the disease can require lifelong blood transfusions. Still, evolution seems to have judged the gene worth keeping - a classic example of the trade-offs built into our biology.
You can find out more on how the antagonistic pleiotropy hypothesis is linked to disease below.
Understanding the disposable soma theory
George Williams’ antagonistic pleiotropy hypothesis - where evolution makes a sort of Faustian bargain - was later expanded by biologist Tom Kirkwood in 1977 with his ”disposable soma” theory.
“Soma” refers to our somatic cells (i.e., all the non-reproductive cells in the body), while “disposable” suggests that once reproduction is achieved, the body becomes biologically expendable.
At first glance, it might seem like Kirkwood was just repeating what Medawar and Williams had already said. But he added an important twist: there is a competition for resources between somatic maintenance and reproductive success. Evolution, Kirkwood argued, allocates energy to where it counts most for gene survival - reproduction, even at the cost of long-term health.
If an organism faces a high risk of dying early from external causes (predators, disease, accidents), it makes sense to invest heavily in staying alive just long enough to reproduce, and not much beyond that.
In general, nature seems to back Kirkwood’s logic. Larger animals, which face fewer external threats, tend to live longer than smaller ones. The most striking example is the bowhead whale, which can live over 200 years - making it the longest-lived mammal on Earth.
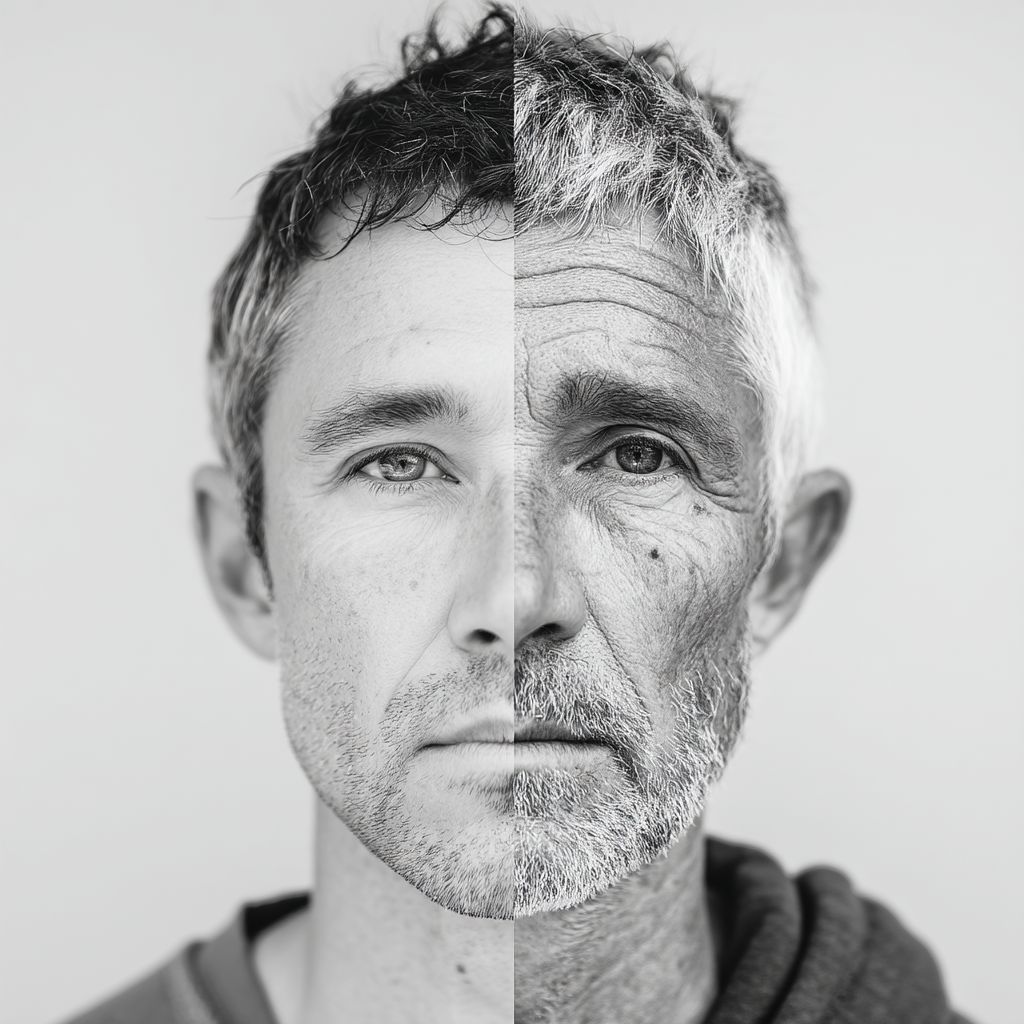
What is the current view of the antagonistic pleiotropy hypothesis (2025)
This trade-off mechanism was theoretical for decades, but it has gained strong empirical support in modern science, making the antagonistic pleiotropy hypothesis highly relevant to current biology and medicine.
Today, antagonistic pleiotropy is recognized as a central theory of aging, alongside complementary ideas like Medawar’s mutation accumulation and Kirkwood’s disposable soma. It is widely accepted that trade-offs between early benefits and late costs are common in biology, and many experts consider antagonistic pleiotropy a ubiquitous phenomenon. For example, Austad and Hoffman note that whenever researchers have looked for these trade-offs, they have found them – suggesting AP effects are perhaps common if not universal across species. As the authors note:
”In the past 20 or so years, molecular biology has presented us with a cornucopia of such genes, and of nine predictions Williams made about senescence and aging, six have proven correct over the last six decades.”
As I alluded to above, not all trade-offs are strictly between reproduction and longevity; beneficial functions in youth might trade off against various aspects of maintenance or health later on. The consensus is that antagonistic pleiotropy helps explain shared molecular mechanisms of aging and points to potential targets for intervention.
In practical terms, the antagonistic pleiotropy hypothesis connects evolutionary biology to medicine, explaining why certain genetic variants associated with diseases persist in populations (they likely gave ancestral advantages). This makes the antagonistic pleiotropy hypothesis highly relevant to understanding age-related diseases and devising interventions today.
Role in Aging Research and Age-Related Diseases
The antagonistic pleiotropy hypothesis is a foundational concept in modern aging research. It underpins numerous experimental findings and guides hypotheses about the aging process. In laboratory studies of short-lived organisms (yeast, worms, flies, mice), many genetic manipulations that extend lifespan often impair early-life functions like fertility or growth, consistent with antagonistic pleiotropy.
For instance, dozens of mutations in C. elegans and Drosophila are known to increase longevity, but when their effects on reproduction or early fitness are measured, trade-offs are frequently observed. Classic experiments in flies showed that selecting for delayed reproduction yields longer-lived lines at the cost of lower early reproduction, directly demonstrating an evolutionary antagonistic pleiotropy trade-off. These findings cement the idea that genetic pathways of aging are intertwined with those of development and reproduction, reflecting evolutionary compromises. Modern gerontology embraces this: many so-called ”longevity genes” (e.g. in insulin/IGF-1 signaling, mTOR, and other growth pathways) likely obey antagonistic pleiotropy, helping young organisms thrive but driving aging later.
Crucially, antagonistic pleiotropy provides insight into age-related diseases. Genes that protect or enhance fitness early in life can predispose individuals to chronic diseases in late life. A striking example is cellular senescence – a tumor-suppressive mechanism: in youth it prevents cancer by halting damaged cells, but in old age those senescent cells accumulate and contribute to tissue degeneration and inflammation (a process called ”inflammaging”).
This has traditionally been considered an antagonistic pleiotropy effect. The p53-driven pathway, central to cancer resistance in youth, has been proposed to promote aging phenotypes later by depleting stem cells and impairing tissue repair. However, recent evidence complicates this picture (which you should expect: this is biology after all): in some contexts, properly regulated p53 activity appears to support longevity by reducing oxidative damage and suppressing tumorigenesis without accelerating aging.
Another well-cited example is the Huntington’s disease gene (HTT) (as I mentioned briefly above): despite causing a lethal neurodegenerative disorder in mid-life, it persisted in humans possibly because it confers a developmental advantage. A 2024 study showed children carrying the mutant HTT gene have better early-life cognitive and motor function and larger brain volumes, but then suffer accelerated decline decades later, aligning with the antagonistic pleiotropy hypothesis.
Similarly, the APOE-ε4 allele, known for increasing Alzheimer’s risk in old age, is hypothesized to have offered benefits for early brain development or pathogen resistance (research is ongoing).
Overall, many alleles associated with diseases (from heart disease to dementia) are being re-examined through the antagonistic pleiotropy lens, revealing that evolutionary ”wins” early in life can become drivers of pathology in our extended post-reproductive years.
Beyond single genes, recent human population studies strongly support the antagonistic pleiotropy model of aging. Large genomic analyses have for the first time documented the predicted trade-offs in people. For example, a December 2023 Science Advances study analyzed data from >276,000 UK Biobank participants: it found that individuals with genetic profiles favoring higher reproductive output tend to have shorter lifespans. There was a negative genetic correlation between reproduction and longevity – essentially, the more one’s genes are tuned for early fertility, the lower the chance of surviving to old age. The study even found that alleles promoting reproduction had been increasing in frequency mid-20th century, implying they were under selection despite shortening life.
Another human study (2025) examined women’s reproductive timing: earlier menarche (puberty) and early childbirth were associated with faster aging and higher risks of age-related diseases later on. Women who started reproduction very early (< age 11 for menarche or <21 for first birth) had significantly higher incidence of conditions like type-2 diabetes, heart failure, and obesity in mid-life – in fact, early puberty nearly doubled the risk of diabetes and quadrupled obesity risk, a clear cost to the early-life benefit of higher fertility.
Genetic analysis in that study identified variants in pathways like IGF-1, mTOR, and AMPK (key growth and metabolic regulators, also mentioned above) connecting early reproductive traits to aging outcomes. This is exactly what antagonistic pleiotropy hypothesis predicts: natural selection favored genes for early reproduction (which might have been advantageous in past environments), but in modern long-lived humans those same pathways drive metabolic disease and aging.
Notably, antagonistic pleiotropy appears to be widespread across many genes and traits, not just reproduction. A 2024 review surveyed known human genetic trade-offs and found over 30 gene variants with well-documented antagonistic pleiotropy effects. These include examples like the sickle-cell mutation (confers malaria resistance early, causes sickle cell disease) and various inflammatory or metabolic genes that protect against infectious disease or famine in youth but contribute to chronic diseases (autoimmune issues, atherosclerosis, etc.) in old age. The prevalence of such examples suggests antagonistic pleiotropy is a common phenomenon in human genetic diversity, with important implications. It helps explain why some ”disease genes” remain common – they likely provided a net fitness advantage in our evolutionary past.
This also serves as a warning for modern medicine: efforts to modify genes or pathways (e.g. via gene therapy or drugs) must consider possible trade-offs. Turning off a gene’s late-life harmful effects could inadvertently disable its beneficial role in youth, and vice versa. Thus, the antagonistic pleiotropy concept is informing precision medicine and longevity research by highlighting that context matters – what is ”good” for the young body may be ”bad” for the old body.

Therapeutic and Clinical Implications
Understanding antagonistic pleiotropy is guiding new approaches to treat aging and age-related diseases. If aging stems partly from genes optimized for early-life fitness, then a tantalizing strategy is to ”tune down” those processes later in life – essentially, reap the early benefits but mitigate the late costs. This is already evident in some anti-aging interventions: for example, the insulin/IGF-1 signaling pathway is vital for growth and reproduction in youth but accelerates aging when hyperactive in later life. Animal studies show that reducing IGF-1 or similar growth signals in adulthood can extend lifespan and healthspan. In mice, overexpression of IGF-1 receptor in the heart yields strong, healthy cardiac function when young but leads to faster cardiac aging and shorter lifespan; conversely, genetically dampening IGF signaling harms youthful performance but preserves cardiac function into old age and extends life. This biphasic outcome exemplifies antagonistic pleiotropy and suggests a therapy:
IGF-1 pathway inhibitors (or caloric restriction mimetics that reduce growth signals) might be given in later life to improve longevity. In fact, the drug rapamycin, which inhibits mTOR (a nutrient-sensing pathway downstream of IGF-1), extends life in lab animals and is thought to work by counteracting growth-related processes that are beneficial early but damaging late. Such geroprotective treatments aim to dial back evolutionary ”thrifty” mechanisms (cell growth, fat storage, etc.) in mid-life to prevent their degenerative consequences.
Another area is senolytic therapies – drugs that clear senescent cells. As mentioned, cellular senescence is antagonistically pleiotropic: it prevents cancer in youth at the cost of aging tissues later. Senolytics attempt to mitigate this late-life cost by removing senescent cells (thereby reducing inflammation and organ decline) without affecting the cancer-protective senescence response earlier in life. Initial trials in animals are promising, reducing frailty and disease in aged mice.
Similarly, immunomodulation is considered: the hyper-vigilant immune activity that fights infection in youth can drive chronic inflammation (inflammaging) in elders, so interventions that re-balance the immune system in old age (e.g. anti-inflammatories or young blood plasma therapies) align with the antagonistic pleiotropy framework.
Antagonistic pleiotropy also urges caution in certain treatments. For example, hormone therapies or growth factor supplements that boost vitality might mimic early-life physiology and thus carry long-term risks. Conversely, completely blocking a pathway that was beneficial for development might cause unwanted side effects. The antagonistic pleiotropy hypothesis encourages targeted or timing-specific interventions – perhaps treating patients in a stage-specific manner.
An illustrative case is the idea of temporarily reducing GH/IGF-1 in adulthood (to extend lifespan) but not during childhood (when it’s needed for development). In cardiovascular medicine, researchers are exploring ways to inhibit IGF-1 or related pathways specifically in aged hearts to treat heart failure without stunting growth in the young. The same logic applies to future gene editing: if we ever disable a gene associated with late-life disease, we must ensure we’re not removing a crucial function that the body needed earlier. In summary, antagonistic pleiotropy has become a conceptual compass for gero medicine and longevity therapeutics, highlighting that effective anti-aging interventions must delicately balance the trade-offs honed by evolution.
Expert Views and Scientific Consensus
What do experts today say about antagonistic pleiotropy and aging? Broadly, the scientific community regards antagonistic pleiotropy as one of the cornerstone theories of why we age. Over the past few years, multiple high-profile studies and reviews have reinforced AP’s validity. In a 2018 review, leading biogerontologists argued that antagonistic pleiotropy effects are ”common, perhaps ubiquitous” in aging biology, and that this implies many aging mechanisms are conserved and potentially targetable. This reflects a growing optimism that identifying antagonistic pleiotropy genes could help combat aging – if those late-life ”penalties” can be alleviated.
Many researchers point out that antagonistic pleiotropy and the related disposable soma theory (which posits the body trades repair for reproduction, also mentioned in more detail above) dovetail nicely: both emphasize resource trade-offs and have gained support from comparative biology (for example, species with high early reproductive output often age faster). As evidence mounts from genomics and experimental evolution, there is an emerging scientific consensus that antagonistic pleiotropy is a real and significant force in aging.
That said, experts also acknowledge complexities. A comprehensive 2024 survey of aging researchers (in PNAS Nexus) found surprising disagreements on foundational principles of aging, including how to interpret evolutionary theories. Some respondents argued that antagonistic pleiotropy might be a general feature of all life, not just a specific cause of senescence. In other words, trade-offs are everywhere – even organisms that don’t ”age” in the typical sense exhibit trade-offs – so a few contend antagonistic pleiotropy alone doesn’t explain aging so much as describe a universal balance. Others question whether antagonistic pleiotropy is sometimes over-invoked, pointing to cases (like certain long-lived mutant mice) where extended lifespan was achieved with minimal reproductive downside.
However, these are seen not as refutations but as nuances: perhaps some organisms find ways around particular trade-offs, or multiple trade-offs exist beyond the obvious fertility vs longevity (for example, trade-offs with stress resistance, growth rate, etc.). Overall, the prevailing view in the field is that Williams’s 1957 antagonistic pleiotropy hypothesis has held up remarkably well.
In summary, the consensus is that antagonistic pleiotropy is a major piece of the aging puzzle, operating in concert with other processes (like late-life mutation accumulation), and it provides a useful framework for both explaining aging’s origin and developing anti-aging strategies.
Recent Research Highlights (2023–2025)
The past few years have seen several important studies that test and apply the antagonistic pleiotropy theory. The table below summarizes some notable recent research and what it tells us about antagonistic pleiotropy in aging and disease:
Year & Source | Study Focus | Key Findings Relevant to Antagonistic Pleiotropy |
---|---|---|
2023 (Sci. Adv.) – Long & Zhang. | Human genomic evidence for AP (UK Biobank study of 276k people) | Found a strong negative genetic correlation between reproductive success and lifespan in humans. |
Huntington’s disease gene (HTT) and brain aging | In young individuals carrying the mutant HTT gene, cognitive and motor performance were better than non-carriers, and brain volumes were larger. But as carriers aged, they underwent steeper neurological decline. | |
Survey of AP in disease-associated alleles | Reviewed literature and identified 30+ human gene variants with well-supported antagonistic pleiotropic effects. | |
Early reproduction vs. aging outcomes (multi-cohort human study) | Showed that women with very early menarche (<11) or first birth (<21) had accelerated aging: significantly higher rates of late-life diseases and lower odds of extreme longevity. |
Takeaway: Recent research clearly supports the utility of the antagonistic pleiotropy framework. From large-scale human genomics to specific genes and pathways, studies are confirming that trade-offs between early-life success and late-life health are real. This not only validates a 60+ year-old theory but also directs today’s scientists and clinicians to consider evolutionary trade-offs when studying aging and treating age-related conditions. The accumulating evidence and expert consensus solidify antagonistic pleiotropy as a vital concept for interpreting aging biology in 2025 and beyond – a reminder that in the story of life, what helps us early can haunt us late, and understanding these dualities is key to unlocking healthier aging.
Enjoyed this deep dive?
🧬 This explainer is part of LEVITY Knowledge - a series exploring the science behind aging and longevity. But there’s more: LEVITY is also a podcast and newsletter covering the latest breakthroughs in life extension, deep-dive features, original essays, and interviews with the scientists and thinkers shaping our future.
👉🏼 Subscribe to LEVITY for weekly insights on longevity biotech and the quest to solve aging.
And remember: Life is good. Death is bad.